Development and Characterization of Gadolinium-Doped Hydroxyapatite to Enhance Biocompatibility in Biomedical Applications
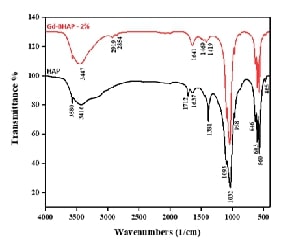
Abstract:
The synthesis and characterization of gadolinium-doped brucinium hydroxyapatite (Gd-BHAP) have garnered significant attention for their potential applications in bone regeneration and dentistry. Hydroxyapatite (HAP) closely resembles the mineral phase of bone, exhibiting biocompatibility, biodegradability, and bioactivity. Hence, this study aims to synthesize and characterize Gd-BHAP to evaluate its potential for enhancing biocompatibility and effectiveness in bone regeneration applications. Gd-BHAP was synthesized via a hydrothermal method using calcium nitrate tetrahydrate and diammonium hydrogen phosphate as precursors, maintaining a calcium-to-phosphate molar ratio of 1:6. Dual dopants, brucine (1%) and gadolinium (+0.5%, 1%, and 2% concentrations), were incorporated, with the pH adjusted to 9. Characterization was performed using XRD, SEM, DLS, FTIR, Fluorescence and UV-Vis spectroscopy. The cytotoxicity of Gd-BHAP was evaluated on Vero cells using the MTT assay across varying concentrations (25–200 µg/mL). Results indicated that the characterization techniques confirmed the successful synthesis of Gd-BHAP, demonstrating rod-shaped morphology and sizes ranging from 70 to 121 nm. FTIR analysis revealed typical absorption bands of hydroxyapatite, while XRD patterns matched known standards for HA, indicating a hexagonal phase. Cytotoxicity results showed a concentration-dependent decrease in Vero cell viability, with 84% viability at 25 µg/mL, decreasing to 73% at 200 µg/mL, indicating acceptable biocompatibility for potential biomedical applications. In conclusion, the synthesized gadolinium-doped brucinium hydroxyapatite exhibits promising characteristics for biomedical applications, particularly in bone regeneration. While higher concentrations may reduce cell viability, the material demonstrates significant biocompatibility, positioning it as a suitable candidate for tissue engineering.References:
[1].
Lara-Ochoa, S., Ortega-Lara, W., &
Guerrero-Beltrán, C. E., 2021. Hydroxyapatite
nanoparticles in drug delivery: physicochemistry and applications. Pharmaceutics, 13(10),
1642.
[2].
Cheng, X., Wei, J., Ge, Q., Xing, D.,
Zhou, X., Qian, Y., & Jiang, G. 2021. The optimized drug delivery systems of treating cancer bone
metastatic osteolysis with nanomaterials. Drug Delivery, 28(1),
37-53.
[3].
De Lama-Odría, M. D. C., Del Valle, L.
J., & Puiggalí, J. 2022. Hydroxyapatite
biobased materials for treatment and diagnosis of cancer. International
journal of molecular sciences, 23(19), 11352.
[4].
Dorozhkin, S. V., 2015.
Calcium orthophosphate-containing biocomposites and hybrid biomaterials for
biomedical applications. Journal of functional biomaterials, 6
(3), 708-832.
[5].
Radha, G., Manjubaashini, N., &
Balakumar, S., 2023. Nano-hydroxyapatite/natural
polymer composite scaffolds for bone tissue engineering: a brief review of
recent trend. In vitro models, 2(5), 125-151.
[6].
Oni, O. P., Hu, Y., Tang, S., Yan, H.,
Zeng, H., Wang, H., & Ran, J. 2023. Syntheses and applications of mesoporous hydroxyapatite: a
review. Materials Chemistry Frontiers, 7(1), 9-43.
[7].
Ielo, I., Calabrese, G., De Luca, G.,
& Conoci, S. 2022. Recent advances in
hydroxyapatite-based biocomposites for bone tissue regeneration in
orthopedics. International journal of molecular sciences, 23(17),
9721.
[8].
Li, L. H., Kim, H. W., Lee, S. H., Kong,
Y. M., & Kim, H. E. 2005. Biocompatibility
of titanium implants modified by microarc oxidation and hydroxyapatite
coating. Journal of Biomedical Materials Research. Part A 73(1),
48-54.
[9].
Turon, P., Del Valle, L.
J., Alemán, C., & Puiggalí, J., 2017. Biodegradable and biocompatible
systems based on hydroxyapatite nanoparticles. Applied Sciences, 7(1),
60.
[10].
Aminzare, M., Eskandari,
A., Baroonian, M. H., Berenov, A., Hesabi, Z. R., Taheri, M., &
Sadrnezhaad, S. K. 2013. Hydroxyapatite nanocomposites: Synthesis, sintering
and mechanical properties. Ceramics International, 39(3),
2197-2206.
[11].
Woodard, J. R.,
Hilldore, A. J., Lan, S. K., Park, C. J., Morgan, A. W., Eurell, J. A. C.,
& Johnson, A. J. W. 2007. The mechanical properties and osteoconductivity
of hydroxyapatite bone scaffolds with multi-scale porosity. Biomaterials, 28(1),
45-54.
[12].
He, P., Sahoo, S., Ng,
K. S., Chen, K., Toh, S. L., & Goh, J. C. H., 2013. Enhanced
osteoinductivity and osteoconductivity through hydroxyapatite coating of
silk‐based tissue‐engineered ligament scaffold. Journal of Biomedical
Materials Research part A, 101(2), 555-566.
[13].
Shi, H., Zhou, Z., Li, W., Fan, Y., Li,
Z., & Wei, J., 2021. Hydroxyapatite based
materials for bone tissue engineering: A brief and comprehensive
introduction. Crystals, 11(2), 149.
[14].
Kang, S., Haider, A., Gupta, K. C., Kim,
H., & Kang, I., 2022. Chemical
bonding of biomolecules to the surface of nano-hydroxyapatite to enhance its
bioactivity. Coatings, 12(7), 999.
[15].
Dutta, S. R., Passi, D., Singh, P.,
& Bhuibhar, A., 2015. Ceramic
and non-ceramic hydroxyapatite as a bone graft material: a brief review. Irish
Journal of Medical Science 184(1), 101-106.
[16].
Lett, J. A., Sagadevan,
S., Fatimah, I., Hoque, M. E., Lokanathan, Y., Léonard, E., & Oh, W. C., 2021.
Recent advances in natural polymer-based hydroxyapatite scaffolds: Properties
and applications. European Polymer Journal, 148,
110360.
[17].
Sprio, S., Dapporto, M., Preti, L.,
Mazzoni, E., Iaquinta, M. R., Martini, F., & Tampieri, A., 2020. Enhancement of the biological and
mechanical performances of sintered hydroxyapatite by multiple ions
doping. Frontiers in Materials, 7, 224.
[18].
Zhao, X., Yang, Z., Liu, Q., Yang, P.,
Wang, P., Wei, S., & Zhao, Z., 2022. Potential load-bearing bone substitution material:
carbon-fiber-reinforced magnesium-doped hydroxyapatite composites with
excellent mechanical performance and tailored biological properties. ACS
Biomaterials Science & Engineering, 8(2), 921-938.
[19].
Fiume, E., Magnaterra, G., Rahdar, A.,
Verné, E., & Baino, F. 2021. Hydroxyapatite for biomedical applications: A short
overview. Ceramics, 4(4), 542-563.
[20].
Radulescu, D. E., Vasile, O. R.,
Andronescu, E., & Ficai, A., 2023. Latest research of doped hydroxyapatite for bone tissue
engineering. International Journal of Molecular Sciences, 24(17),
13157.
[21].
Du, M., Chen, J., Liu, K., Xing, H.,
& Song, C., 2021. Recent advances in biomedical
engineering of nano-hydroxyapatite including dentistry, cancer treatment and
bone repair. Composites Part B: Engineering, 215,
108790.
[22].
Mondal, S., Park, S.,
Choi, J., Vo, T. M. T., Shin, J. H., Kang, Y. H., & Oh, J., 2020. Rare
earth element doped hydroxyapatite luminescent bioceramics contrast agent for
enhanced biomedical imaging and therapeutic applications. Ceramics
International, 46(18), 29249-29260.
[23].
Gu, M., Li, W., Jiang, L., & Li, X.,
2022. Recent progress of rare
earth doped hydroxyapatite nanoparticles: Luminescence properties, synthesis
and biomedical applications. Acta Biomaterialia, 148,
22-43.
[24].
Somoza, M., Rial, R., Liu, Z., Llovo, I.
F., Reis, R. L., Mosqueira, J., & Ruso, J. M., 2023. Microfluidic fabrication of gadolinium-doped
hydroxyapatite for theragnostic applications. Nanomaterials, 13(3),
501.
[25].
Uskoković, V., 2015. The
role of hydroxyl channel in defining selected physicochemical peculiarities
exhibited by hydroxyapatite. RSC advances, 5(46),
36614-36633.
[26].
Mushtaq, A., Zhao, R.,
Luo, D., Dempsey, E., Wang, X., Iqbal, M. Z., & Kong, X., 2021. Magnetic
hydroxyapatite nanocomposites: The advances from synthesis to biomedical
applications. Materials & Design, 197, 109269.
[27].
Lv, Y., Shi, Q., Jin, Y., Ren, H., Qin,
Y., Wang, B., & Song, S., 2018. Preparation and Luminescent Properties of the antibacterial
materials of the La3+ Doped Sm3+-Hydroxyapatite. In Journal of Physics:
Conference Series 986(1)
[28].
Kim, H. K., Lee, G. H., & Chang, Y.,
2018. Gadolinium as an MRI
contrast agent. Future Medicinal Chemistry, 10(6),
639-661.
[29].
Fatima, A., Ahmad, M.
W., Al Saidi, A. K. A., Choudhury, A., Chang, Y., & Lee, G. H., 2021.
Recent advances in gadolinium based contrast agents for bioimaging
applications. Nanomaterials, 11(9), 2449.
[30].
Grobner, T., & Prischl, F. C., 2007.
Gadolinium and
nephrogenic systemic fibrosis. Kidney international, 72(3),
260-264.
[31].
Wei, M., Evans, J. H., Bostrom, T.,
& Grøndahl, L., 2003. Synthesis
and characterization of hydroxyapatite, fluoride-substituted hydroxyapatite and
fluorapatite. Journal of materials science: Materials in medicine, 14(4),
311-320.
[32].
Rapacz-Kmita, A.,
Paluszkiewicz, C., Ślósarczyk, A., & Paszkiewicz, Z., 2005. FTIR and XRD
investigations on the thermal stability of hydroxyapatite during hot pressing
and pressureless sintering processes. Journal of Molecular Structure, 744,
653-656.
[33].
Shaltout, A. A., Allam,
M. A., & Moharram, M. A., 2011. FTIR spectroscopic, thermal and XRD
characterization of hydroxyapatite from new natural sources. Spectrochimica
Acta Part A: Molecular and Biomolecular Spectroscopy, 83(1),
56-60.
[34].
Abifarin, J. K., Obada,
D. O., Dauda, E. T., & Dodoo-Arhin, D., 2019. Experimental data on the
characterization of hydroxyapatite synthesized from biowastes. Data in
brief, 26, 104485.
[35].
Zhang, C., Yang, J.,
Quan, Z., Yang, P., Li, C., Hou, Z., & Lin, J., 2009. Hydroxyapatite
nano-and microcrystals with multiform morphologies: controllable synthesis and
luminescence properties. Crystal Growth and Design, 9(6),
2725-2733.
[36].
Marković, S.,
Veselinović, L., Lukić, M. J., Karanović, L., Bračko, I., Ignjatović, N., &
Uskoković, D., 2011. Synthetical bone-like and biological hydroxyapatites: a
comparative study of crystal structure and morphology. Biomedical
Materials, 6(4), 045005.
[37].
Chandrasekar,
A., Sagadevan, S., & Dakshnamoorthy, A., 2013. Synthesis and
characterization of nano-hydroxyapatite (n-HAP) using the wet chemical
technique. Int. J. Phys. Sci, 8(32),
1639-1645.
[38].
Montalbano, G.,
Molino, G., Fiorilli, S., & Vitale-Brovarone, C., 2020. Synthesis and
incorporation of rod-like nano-hydroxyapatite into type I collagen matrix: A
hybrid formulation for 3D printing of bone scaffolds. Journal of the European Ceramic Society, 40(11), 3689-3697.
[39].
Machla,
F., Sokolova, V., Platania, V., Prymak, O., Kostka, K., Kruse, B., &
Bakopoulou, A., 2023. Tissue engineering at the dentin-pulp interface using
human treated dentin scaffolds conditioned with DMP1 or BMP2 plasmid
DNA-carrying calcium phosphate nanoparticles. Acta Biomaterialia, 159, 156-172.
[40].
Nenad
L. Ignjatović, Lidija Mančić, MarinaVuković, Zoran Stojanović, MarkoG. Nikolić,
Srečo Škapin, Sonja Jovanović, LjiljanaVeselinović, Vuk Uskoković, Snežana
Lazić, Smilja Marković, Miloš M. Lazarević & Dragan P. Uskoković, 2019.
Rare-earth (Gd3+, Yb3+/Tm3+, Eu3+)
co-doped hydroxyapatite as magnetic, up-conversion and down-conversion
materials for multimodal imaging. Scientific reports, 9:16305
[41].
James J. Hagan, Susan
Cicero Taylor, and Michael F. Tweedle 1988. Fluorescence Detection of
Gadolinium Chelates Separated by Reversed-Phase High-Performance Liquid
Chromatography, American Chemical Society, 60, 514-516.
[42].
Doan,
V. H. M., Vu, D. D., Mondal, S., Vo, T. M. T., Ly, C. D., Nguyen, V. T., &
Oh, J., 2023. Yb–Gd Codoped Hydroxyapatite as a Potential Contrast Agent for
Tumor-Targeted Biomedical Applications. ACS
Biomaterials Science & Engineering, 9 (8), 4607-4618.
[43].
Minh,
D. V. H., 2022. Biomedical instruments design and development focused on
ultrasound, fluorescence, and photoacoustic imaging-guided therapeutic
modalities, Doctoral dissertation, 부경대학교.
[44].
Sha, S., Zhang, L.,
Liu, H., Chen, J., Che, Y., Zhang, F., & Song, C., 2021. Synthesis and
visible-light photocatalytic degradation of Ag 3 PO 4/AgBr/hydroxyapatite
ternary nanocomposites prepared from oyster shells. RSC advances, 11(26), 15598-15607.
[45].
Kpemissi, M.,
Eklu-Gadegbeku, K., Veerapur, V. P., Potârniche, A. V., Adi, K., Vijayakumar,
S., Banakar, S. M., Thimmaiah, N. V., Metowogo, K., & Aklikokou, K., 2019.
Antioxidant and nephroprotection activities of Combretum micranthum: A
phytochemical, in-vitro and ex-vivo studies. Heliyon, 5(3), e01365.
[46].
Do Amaral, M. B.,
Viana, R. B., Viana, K. B., Diagone, C. A., Denis, A. B., & de Guzzi
Plepis, A. M., 2020. In
vitro and in vivo response of composites based on chitosan, hydroxyapatite and
collagen. Acta Scientiarum. Technology,
42.