Polysaccharides in Organ Fibrosis: Therapeutic and Preventive Effects Through Gut Microbiota Modulation
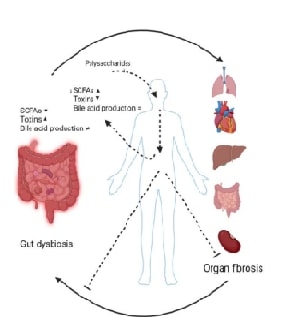
Abstract:
Fibrosis is characterized by the proliferation of
fibrous tissue and decreased functional cells within the affected organs,
ultimately resulting in organ damage or failure. The incidence of this
pathological process is on the rise globally, necessitating the development of
more efficient therapeutic interventions. Recent scientific investigations have
put forth the idea that polysaccharides extracted from natural sources exhibit
promising abilities in alleviating fibrotic conditions by mitigating inflammatory
responses and influencing intestinal microbiota composition. This scholarly
discourse delves into the intricate relationship between polysaccharides and
organ fibrosis concerning the dynamics of the intestinal microbiota, offering
profound insights that could significantly influence the direction of future endeavours
in drug development and treatment modalities. The in-depth exploration of these
interconnected factors holds immense potential in shaping innovative strategies
to combat fibrosis-related disorders and enhance patient outcomes in a clinical
setting. The potential impact of this research on future drug development and
treatment modalities cannot be overstated, as it underscores the importance of
the study and its potential to influence the direction of future research in
the field.
References:
[2].
Cheong,
K.-L., Yu, B., Teng, B., Veeraperumal, S., Xu, B., et al., 2023, Post-COVID-19
syndrome management: Utilizing the potential of dietary polysaccharides, Biomedicine
& Pharmacotherapy, 166, 115320. https://doi.org/10.1016/j.biopha.2023.115320
[3].
Jayaseelan,
V. P., 2019, In silico validation of the non-antibiotic drugs acetaminophen and
ibuprofen as antibacterial agents against red complex pathogens, Journal of
Periodontology, 90, 1441-1448. https://doi.org/10.1002/JPER.18-0673. https://doi.org/10.1016/j.sajb.2022.03.015
[4].
Wang,
M., Veeraperumal, S., Zhong, S., Cheong, K.-L., 2023, Fucoidan-derived
functional oligosaccharides: Recent developments, preparation, and potential
applications, Foods, 12, 878. https://doi.org/10.3390/foods12040878
[5].
Corrie,
L., Gulati, M., Awasthi, A., Vishwas, S., Kaur, J., et al., 2022,
Polysaccharide, fecal microbiota, and curcumin-based novel oral colon-targeted
solid self-nanoemulsifying delivery system: Formulation, characterization, and
in-vitro anticancer evaluation, Materials Today Chemistry, 26, 101165. https://doi.org/10.1016/j.mtchem.2022.101165
[6].
Zhang,
A., Wang, J., Hu, Y., Qiu, Y., Dong, C., 2024, Polysaccharides play an
anti-fibrotic role by regulating intestinal flora: A review of research
progress, International Journal of Biological Macromolecules, 131982. https://doi.org/10.1016/j.ijbiomac.2024.131982
[7].
Bhat,
A.A., Gupta, G., Alharbi, K.S., Afzal, O., Altamimi, A.S.A., et al., 2022,
Polysaccharide-Based Nanomedicines Targeting Lung Cancer, Pharmaceutics,
14, 2788. https://doi.org/10.3390/ pharmaceutics14122788
[8].
Ren,
Q., Zhang, L.-y., Li, Z., 2020, Advances in the research of anti-organ fibrosis
drugs, Acta Pharmaceutica Sinica, 2510–28.
[9].
Zhao,
X., Chen, J., Sun, H., Zhang, Y., Zou, D., 2022, New insights into fibrosis
from the ECM degradation perspective: the macrophage-MMP-ECM interaction, Cell
& Bioscience, 12, 117. https://doi.org/10.1186/s13578-022-00856-w
[10]. Rockey, D. C., Bell, P. D., Hill, J. A.,
2015, Fibrosis—a common pathway to organ injury and failure, New England
Journal of Medicine, 372, 1138–1149. DOI: 10.1056/NEJMra1300575
[11]. Zhao, M., Wang, L., Wang, M., Zhou, S., Lu,
Y., et al., 2022, Targeting fibrosis: Mechanisms and clinical trials, Signal
Transduction and Targeted Therapy, 7, 206. https://doi.org/10.1038/s41392-022-01070-3
[12]. Kozawa, S., Tejima, K., Takagi, S., Kuroda,
M., Nogami-Itoh, M., et al., 2023, Latent inter-organ mechanism of idiopathic
pulmonary fibrosis unveiled by a generative computational approach, Scientific
Reports, 13, 21981. https://doi.org/10.1038/s41598-023-49281-0
[13]. Álvarez, J., Real, J. M.
F., Guarner, F., Gueimonde, M., Rodríguez, J. M., et al., 2021, Microbiota
intestinal y salud, Gastroenterologíay Hepatología, 44, 519–535. 10.1016/j.gastrohep.2021.01.009.
[14]. Wu, Y., Li, Y., Luo, Y., Zhou, Y., Wen, J.,
et al., 2022, Gut microbiome and metabolites: The potential key roles in
pulmonary fibrosis, Frontiers in Microbiology, 13, 943791. https://doi.org/10.3389/fmicb.2022.943791
[15]. Drakopanagiotakis, F., Stavropoulou, E.,
Tsigalou, C., Nena, E., Steiropoulos, P., 2022, The role of the microbiome in
connective-tissue-associated interstitial lung disease and pulmonary
vasculitis, Biomedicines, 10, 3195. https://doi.org/10.3390/biomedicines10123195
[16]. Chioma, O. S., Mallott, E. K., Chapman, A.,
Van Amburg, J. C., Wu, H., et al., 2022, Gut microbiota modulates lung fibrosis
severity following acute lung injury in mice, Communications Biology, 5,
1401. https://doi.org/10.1038/s42003-022-04357-x
[17]. Aydın, M. M., Akçalı, K. C., 2018, Liver
fibrosis, The Turkish Journal of Gastroenterology, 29, 14. https://doi.org/10.5152/tjg.2018.17330
[18]. Jayaseelan, V. P., Arumugam, P., 2019,
Dissecting the theranostic potential of exosomes in autoimmune disorders,
Cellular Molecular Immunology, 16, 935-936.
https://doi.org/10.1038/s 41423-019-0310-5
Friedman, S. L., 2024, Hepatic fibrosis and cancer: the silent threats of
metabolic syndrome, Diabetes & Metabolism Journal, 48, 161. https://doi.org/10.4093/dmj.2023.0240
[19]. Lee, C.-m., Yoon, E. L., Kim, M., Kang,
B.-K., Cho, S., et al., 2024, Prevalence, distribution, and hepatic fibrosis
burden of the different subtypes of steatotic liver disease in primary care
settings, Hepatology, 79, 1393–1400. https://doi.org/10.1097/hep.0000000000000664
[20]. Gao, L.-L., Ma, J.-M., Fan, Y.-N., Zhang,
Y.-N., Ge, R., et al., 2021, Lycium barbarum polysaccharide combined with
aerobic exercise ameliorated nonalcoholic fatty liver disease through restoring
gut microbiota, intestinal barrier and inhibiting hepatic inflammation,
International Journal of Biological Macromolecules, 183, 1379–1392. https://doi.org/10.1016/j.ijbiomac.2021.05.066
[21]. Li, S., 2022, Investigation of the Anti-HF
Mechanism of Taraxacum mongolicum Polysaccharide with Astragalus
Polysaccharide. https://doi.org/10.1016/j.ijbiomac.2023.129193
[22]. Shu, Y., Huang, Y., Dong, W., Fan, X., Sun,
Y., et al., 2023, The polysaccharides from Auricularia auricula alleviate
non-alcoholic fatty liver disease via modulating gut microbiota and bile acids
metabolism, International Journal of Biological Macromolecules, 246,
125662. https://doi.org/10.1016/j.ijbiomac.2023.125662
[23]. Han, C., Li, Z., Liu, R., Zhao, Z., Wang,
Y., et al., 2023, Lonicerae flos polysaccharides improve nonalcoholic fatty
liver disease by activating the adenosine 5′-monophosphate-activated protein
kinase pathway and reshaping gut microbiota, Journal of the Science of Food
and Agriculture, 103, 7721–7738. https://doi.org/10.1002/jsfa.12854
[24]. Fang, S., Wang, T., Li, Y., Xue, H., Zou,
J., et al., 2022, Gardenia jasminoides Ellis polysaccharide ameliorates
cholestatic liver injury by alleviating gut microbiota dysbiosis and inhibiting
the TLR4/NF-κB signaling pathway, International Journal of Biological
Macromolecules, 205, 23–36. https://doi.org/10.1016/j.ijbiomac.2022.02.056
[25]. Humphreys, B. D., 2018, Mechanisms of renal
fibrosis, Annual Review of Physiology, 80, 309–326. https://doi.org/10.1146/annurev-physiol-022516-034227
[26]. Liu, Y., 2011, Cellular and molecular
mechanisms of renal fibrosis, Nature Reviews Nephrology, 7, 684–696. https://doi.org/10.1038/nrneph.2011.149
[27]. Nogueira, A., Pires, M. J., Oliveira, P.
A., 2017, Pathophysiological mechanisms of renal fibrosis: a review of animal
models and therapeutic strategies, In Vivo, 31, 1–22. https://doi.org/10.21873/invivo.11019
[28]. Yang, J., Dong, H., Wang, Y., Jiang, Y.,
Zhang, W., et al., 2020, Cordyceps cicadae polysaccharides ameliorated renal
interstitial fibrosis in diabetic nephropathy rats by repressing inflammation
and modulating gut microbiota dysbiosis, International Journal of Biological
Macromolecules, 163, 442–456. https://doi.org/10.1016/j.ijbiomac.2020.06.153
[29]. Zhang, M., Yang, L., Zhu, M., Yang, B.,
Yang, Y., et al., 2022, Moutan cortex polysaccharide ameliorates diabetic
kidney disease via modulating gut microbiota dynamically in rats, International
Journal of Biological Macromolecules, 206, 849–860. https://doi.org/10.1016/j.ijbiomac.2022.03.077
[30]. Feng, Y., Weng, H., Ling, L., Zeng, T.,
Zhang, Y., et al., 2019, Modulating the gut microbiota and inflammation is
involved in the effect of Bupleurum polysaccharides against diabetic
nephropathy in mice, International Journal of Biological Macromolecules,
132, 1001–1011. https://doi.org/10.1016/j.ijbiomac.2019.03.242
[31]. Liu, J.-X., Yuan, H.-Y., Li, Y.-N., Wei,
Z., Liu, Y., Liang, J., 2022, Ephedra sinica polysaccharide alleviates airway
inflammations of mouse asthma-like induced by PM2.5 and ovalbumin via the
regulation of gut microbiota and short chain fatty acid, Journal of Pharmacy
and Pharmacology, 74, 1784–96. https://doi.org/10.1093/jpp/rgac078
[32]. Shi, C., Zhou, L., Li, H., Shi, X., Zhang,
Y., et al., 2022, Intestinal microbiota metabolizing Houttuynia cordata
polysaccharides in H1N1 induced pneumonia mice contributed to Th17/Treg
rebalance in gut-lung axis, International Journal of Biological
Macromolecules, 221, 288–302. https://doi.org/10.1016/j.ijbiomac.2022.09.015