A Novel Study Elucidating the Effects of Glucose and Static Magnetic Field on Osteoblast Differentiation Markers
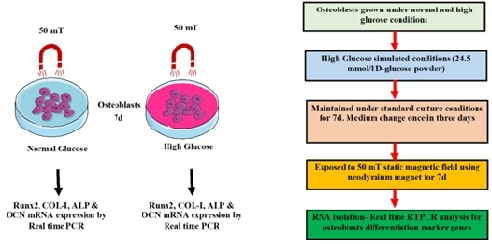
Abstract:
In this
study, we explored the expression dynamics of osteoblast differentiation marker
genes under the influence of static magnetic fields (SMF) in high glucose conditions.
High glucose can significantly impair osteoblast function and differentiation-
particularly in diabetic patients with persistent hyperglycemia. In the in-vivo
diabetic-like environment that is under glucose level, SMF’s role in bone
formation was evaluated using Human osteoblastic cells that were treated with
SMF for up to 7 days. The expression of key osteogenic markers such as alkaline phosphatase (ALP), osteocalcin (OCN), and
collagen type I (COL1A1) was analysed using qRT-PCR and ELISA. The findings
demonstrated that high glucose led to pronounced inhibition of osteoblast
differentiation markers. Conversely, being exposed to an SMF dramatically
increases the expression of markers for osteoblast differentiation.
Additionally, the inhibitory effects of glucose on osteoblast development
appear to be lessened upon SMF exposure. These results emphasize the
significance of taking into account the positive effects of SMF, which may have
therapeutic potential in bone repair.
References:
[1]. Li, M., Chi, X., Wang, Y.,
Setrerrahmane, S., Xie, W., Xu, H., 2022, Trends in insulin resistance:
insights into mechanisms and therapeutic strategy, Signal Transduct Target
Ther, vol. 7, no. 1, p. 216.
[2]. Bao, K., Jiao, Y., Xing, L., Zhang,
F., Tian, F., 2023, The role of Wnt signaling in diabetes‐induced osteoporosis,
Diabetol Metab Syndr, vol. 15, no. 1, p. 84.
[3]. Ross, B. J., Lee, O. C., Harris, M. B.,
Dowd, T. C., Savoie, F. H. 3rd, Sherman, W. F., 2022, The impact of diabetes on
osteoporosis management and secondary fracture risk after primary fragility
fractures: a propensity score-matched cohort study, J Am Acad Orthop Surg,
vol. 30, no. 2, p. e204–12.
[4]. de Paula, F. J. A., Horowitz, M. C.,
Rosen, C. J., 2010, Novel insights into the relationship between diabetes and
osteoporosis, Diabetes Metab Res Rev, vol. 26, no. 8, p. 622–30.
[5]. Gil-Díaz, M. C., Raynor, J., O’Brien,
K. O., Schwartz, G. J., Weber, D. R., 2019, Systematic review: associations of
calcium intake, vitamin D intake, and physical activity with skeletal outcomes
in people with type 1 diabetes mellitus, Acta Diabetol, vol. 56, no. 10, p.
1091–102.
[6]. Yang, J., Ma, C., Zhang, M., 2019,
High glucose inhibits osteogenic differentiation and proliferation of MC3T3‑E1
cells by regulating P2X7, Mol Med Rep, vol. 20, no. 6, p. 5084–90.
[7]. Zhang, J., Ding, C., Ren, L., Zhou,
Y., Shang, P., 2014, The effects of static magnetic fields on bone, Prog
Biophys Mol Biol, vol. 114, no. 3, p. 146–52.
[8]. Yang, T. C., Maeda, Y., Gonda, T.,
Wada, M., 2013, Magnetic attachment for implant overdentures: influence of
contact relationship with the denture base on stability and bending strain, Int
J Prosthodont, vol. 26, no. 6, p. 563–5.
[9]. Aksu, A. E., Dursun, E., Calis, M.,
Ersu, B., Safak, T., Tözüm, T. F., 2014, Intraoral use of extraoral implants
for oral rehabilitation of a pediatric patient after resection of Ewing sarcoma
of the mandible and reconstruction with iliac osteocutaneous free flap, J
Craniofac Surg, vol. 25, no. 3, p. 930–3.
[10]. Siadat, H., Bassir, S. H., Alikhasi,
M., Shayesteh, Y. S., Khojasteh, A., Monzavi, A., 2012, Effect of static
magnetic fields on the osseointegration of immediately placed implants: a
randomized controlled clinical trial, Implant Dent, vol. 21, no. 6, p. 491–5.
[11]. Leesungbok, R., Ahn, S. J., Lee, S. W.,
Park, G. H., Kang, J. S., Choi, J. J., 2013, The effects of a static magnetic
field on bone formation around a sandblasted, large-grit, acid-etched–treated
titanium implant, J Oral Implantol, vol. 39, no. S1, p. 248–55.
[12]. Cipriani, C., Colangelo, L.,
Santori, R., Renella, M., Mastrantonio, M., Minisola, S., et al., 2020, The
interplay between bone and glucose metabolism, Front Endocrinol, vol. 11, p.
122.
[13]. Song, F., Lee, W. D., Marmo, T., Ji,
X., Song, C., Liao, X., et al., 2023, Osteoblast-intrinsic defect in glucose
metabolism impairs bone formation in type II diabetic mice, bioRxiv, p.
10.1101/2023.01.16.524248.
[14]. Mannucci, E., Monami, M., 2017, Bone
fractures with sodium-glucose co-transporter-2 inhibitors: how real is the
risk?, Drug Saf, vol. 40, no. 2, p. 115–9.
[15]. Tang, H. L., Li, D. D., Zhang, J. J.,
Hsu, Y. H., Wang, T. S., Zhai, S. D., et al., 2016, Lack of evidence for a
harmful effect of sodium-glucose co-transporter 2 (SGLT2) inhibitors on
fracture risk among type 2 diabetes patients: a network and cumulative
meta-analysis of randomized controlled trials, Diabetes Obes Metab, vol. 18,
no. 12, p. 1199–206.
[16]. Lv, H., Wang, Y., Zhen, C., Liu, J.,
Chen, X., Zhang, G., et al., 2023, A static magnetic field improves bone
quality and balances the function of bone cells with regulation on iron
metabolism and redox status in type 1 diabetes, FASEB J, vol. 37, no. 7, p.
e22985.
[17]. Vimalraj, S., Sekaran, S., 2023,
RUNX family as a promising biomarker and a therapeutic target in bone cancers:
a review on its molecular mechanisms behind tumorigenesis, Cancers, vol.
15, no. 12, p. 3247.
[18]. Jonason, J. H., Xiao, G., Zhang, M.,
Xing, L., Chen, D., 2009, Post-translational regulation of Runx2 in bone and
cartilage, J Dent Res, vol. 88, no. 8, p. 693–703.
[19]. Sekaran, S., Vimalraj, S.,
Thangavelu, L., 2021, The physiological and pathological role of tissue
nonspecific alkaline phosphatase beyond mineralization, Biomolecules, vol. 11,
no. 11.
[20]. Amirrah, I. N., Lokanathan, Y.,
Zulkiflee, I., Wee, M. F. M. R., Motta, A., Fauzi, M. B., 2022, A comprehensive
review on collagen type I development of biomaterials for tissue engineering:
from biosynthesis to bioscaffold, Biomedicines, vol. 10, no. 9.
[21]. Selvaraj, V., Sekaran, S.,
Dhanasekaran, A., Warrier, S., 2024, Type 1 collagen: synthesis, structure, and
key functions in bone mineralization, Differentiation, p. 100757.
[22]. Saito, M., Kida, Y., Kato, S.,
Marumo, K., 2014, Diabetes, collagen, and bone quality, Curr Osteoporos Rep,
vol. 12, no. 2, p. 181–8.
[23]. Zaitseva, O. V., Shandrenko, S. G.,
Veliky, M. M., 2015, Biochemical markers of bone collagen type I metabolism, Ukr
Biochem J, vol. 87, no. 1, p. 21–32.
[24]. Kim, E. C., Park, J., Kwon, I. K.,
Lee, S. W., Park, S. J., Ahn, S. J., 2017, Static magnetic fields promote
osteoblastic/cementoblastic differentiation in osteoblasts, cementoblasts, and
periodontal ligament cells, J Periodontal Implant Sci, vol. 47, no. 5,
p. 273–91.
[25]. Vimalraj, S., Govindarajan, D.,
Sudhakar, S., Suresh, R., Palanivel, P., Sekaran, S., 2024, Chitosan-derived
chito-oligosaccharides promote osteoblast differentiation and offer
anti-osteoporotic potential: molecular and morphological evidence from a zebrafish
model, Int J Biol Macromol, vol. 259, p. 129250.
[26]. Govindarajan, D., Saravanan, S., Sudhakar, S., Vimalraj, S., 2023, Graphene: a multifaceted carbon-based material for bone tissue engineering applications, ACS Omega, vol. 9, no. 1, p. 67–80.