Influence of Acid and Alkali Surface Modifications on Titanium Implants: Enhancing Osseointegration and Osteoblast Differentiation
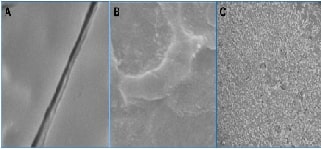
Abstract:
Titanium implants are widely used in biomedical
applications due to their excellent biocompatibility and mechanical properties.
However, achieving optimal osseointegration remains a challenge. Surface
modification techniques, such as acid etching and alkali etching, have been
shown to improve implant surface properties, including roughness and chemistry,
thereby enhancing cellular adhesion and modulating molecular pathways critical
for bone formation. This study evaluated titanium implant surfaces modified using
acid etching and alkali etching. Surface topographies were characterized using
scanning electron microscopy (SEM), revealing distinct morphologies.
Acid-etched surfaces exhibited uniformly roughened structures, while
alkali-etched surfaces showed smoother textures with pits. Chemical composition
analysis, performed using X-ray photoelectron spectroscopy (XPS), indicated
significant alterations, including the formation of bioactive layers that
enhance implant integration. In vitro experiments demonstrated that acid-etched
surfaces significantly promoted osteoblast adhesion and differentiation
compared to alkali-etched surfaces. This was supported by the upregulation of
osteogenic molecular markers such as Runx2, SP7, and DLX5, which are vital for
bone formation. These findings suggest that acid etching enhances the
biological performance of titanium implants, facilitating cellular behaviours
necessary for successful osseointegration. In conclusion, acid etching and
alkali etching are effective methods for improving titanium implant surfaces,
with acid-etched surfaces showing superior potential in promoting osteoblast
differentiation and adhesion. Further research is needed to investigate the
long-term clinical impact of these surface modifications to optimize implant
success and durability.
References:
[1]. Sivakumar, N. K., Palaniyappan, S., Sekar, V., Alodhayb, A., &
Braim, M. 2023. An optimization approach for studying the effect of lattice
unit cell’s design-based factors on additively manufactured poly methyl
methacrylate cranio-implant. Journal of the Mechanical Behavior of
Biomedical Materials, 141(105791), 105791. https://doi.org/10.1016/j.jmbbm.2023.105791
[2]. Patil, S., Bhandi, S., Alzahrani, K. J., Alnfiai, M. M., Testarelli, L.,
Soffe, B. W., Licari, F. W., Awan, K. H., & Tanaka, E. 2023. Efficacy of
laser in re-osseointegration of dental implants-a systematic review. Lasers
in Medical Science, 38(1), 199. https://doi.org/10.1007/s10103-023-03860-9
[3]. Madhu, K., Kannan, S., Perumal, A., & Shanmugam, P.
2023. Biofunctionalized nanocomposite coating on Cp-titanium
with reduce implant failures. Vacuum, 215(112328), 112328.
https://doi.org/10.1016/j.vacuum.2023.112328
[4]. Raval, A., S Yadav, N., Narwani, S., Somkuwar, K., Verma, V., Almubarak,
H., Alqahtani, S. M., Tasleem, R., Luke, A. M., Kuriadom, S. T., &
Karobari, M. I. 2023. Antibacterial efficacy and surface characteristics of
boron nitride coated dental implant: An in-vitro study. Journal of
Functional Biomaterials,ss 14(4). https://doi.org/10.3390/jfb14040201
[5]. Duraisamy, R., Ganapathy, D. and Shanmugam, R., 2021.
Applications of chitosan in dental implantology-A literature review. Int.
J. Dent. Oral Sci, 8, pp.4140-4146.
https://doi.org/10.19070/2377-8075-21000846
[6]. Groner, Y., Ito, Y., Liu, P., Neil, J. C., Speck, N. A., & van
Wijnen, A. 2017. RUNX Proteins in Development and Cancer. Springer. https://play.google.com/store/books/details?id=1pNcDgAAQBAJ
[7]. Biosynthesis of Vitex-negundo mineralized hydroxyapatite coating on Ti
for implant applications. (n.d.).
[8]. Liu, L., Luo, P., Liao, H., Yang, K., Yang, S., & Tu,
M., 2024. Effects of aligned PLGA/SrCSH composite scaffolds on
in vitro growth and osteogenic differentiation of human mesenchymal stem cells.
Journal of Biomedical Materials Research. Part B, Applied Biomaterials, 112(1),
e35366. https://doi.org/10.1002/jbm.b.35366
[9]. Pederson, E. D., Lamberts, B. L., NAVAL DENTAL RESEARCH INST GREAT LAKES
IL., & Naval Dental Research Institute (U.S.)., 1989. Effect of
Root-Surface Modification of Human Teeth on Adherence of Fibronectin. https://books.google.com/books/about/Effect_of_Root_Surface_Modification_of_H.html?hl=&id=xujdNwAACAAJ
[10]. Adden, N., 2006. Modification of Titanium to Generate Biocompatible
and Bioactive Implant Surfaces. https://books.google.com/books/about/Modification_of_Titanium_to_Generate_Bio.html?hl=&id=OAoBPwAACAAJ
[11]. Kim, K.-H., Narayanan, R., & Rautray, T. R., 2010. Surface
Modification of Titanium for Biomaterial Applications. https://books.google.com/books/about/Surface_Modification_of_Titanium_for_Bio.html?hl=&id=Ii0cQgAACAAJ
[12]. Omoniala, K., 2016. Surface Modification Strategies for Antimicrobial
Titanium Implant Materials with Enhanced Osseointegration. https://books.google.com/books/about/Surface_Modification_Strategies_for_Anti.html?hl=&id=cjcbvwEACAAJ
[13]. Abdulla, M. A., Hasan, R. H., & Al-Hyani, O. H. 2023. Impact of
Er,Cr:YSGG Laser, Sandblast, and Acid Etching Surface Modification on Surface
Topography of Biodental Titanium Implants. Journal of Lasers in Medical
Sciences, 14, e38. https://doi.org/10.34172/jlms.2023.38
[14]. Baima, G., Romano, F., Roato, I., Mosca Balma, A.,
Pedraza, R., Faga, M. G., Amoroso, F., Orrico, C., Genova, T., Aimetti, M.,
& Mussano, F. 2024. Efficacy of a Solution
Containing 33% Trichloroacetic Acid and Hydrogen Peroxide in Decontaminating
Machined vs. Sand-Blasted Acid-Etched Titanium Surfaces. Journal of
Functional Biomaterials, 15(1). https://doi.org/10.3390/jfb15010021
[15]. Ochi, Y., Yoshida, K., Huang, Y.-J., Kuo, M.-C., Nannya, Y., Sasaki, K.,
Mitani, K., Hosoya, N., Hiramoto, N., Ishikawa, T., Branford, S.,
Shanmuganathan, N., Ohyashiki, K., Takahashi, N., Takaku, T., Tsuchiya, S.,
Kanemura, N., Nakamura, N., Ueda, Y., … Shih, L.-Y., 2021. Clonal evolution and
clinical implications of genetic abnormalities in blastic transformation of
chronic myeloid leukaemia. Nature Communications, 12(1), 2833.
https://doi.org/10.1038/s41467-021-23097-w
[16]. Albrektsson, T., Tengvall, P., Amengual, L., Coli, P., Kotsakis, G. A.,
& Cochran, D. 2022. Osteoimmune regulation underlies oral implant
osseointegration and its perturbation. Frontiers in Immunology, 13,
1056914. https://doi.org/10.3389/fimmu.2022.1056914
[17]. Li, H. 2007. Functional Studies of Dlx5 During Bone Formation:
Implications of Dlx Genes in Promoting Osteoblast Differentiation. https://books.google.com/books/about/Functional_Studies_of_Dlx5_During_Bone_F.html?hl=&id=iHXezQEACAAJ
[18]. Liao, T., Xu, X., Wu, J., Xie, Y., & Yan, J. 2023. Increased
expression levels of DLX5 inhibit the development of the nervous system. International
Journal of Developmental Neuroscience: The Official Journal of the
International Society for Developmental Neuroscience, 83(8),
728–739. https://doi.org/10.1002/jdn.10300