Computational Design of Bioactive Epigallocatechin Gallate (EGCG) Analogues Targeting Heme Oxygenase-1 (HO-1) Pathway for Metabolic Regulation
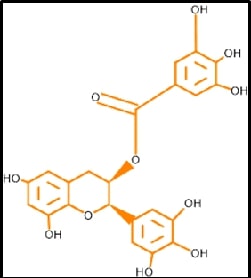
Abstract:
Epigallocatechin-gallate
(EGCG) is a flavone-based natural product that has a more significant impact on
Diabetes and other cardiometabolic complications. This in silico based
computer-aided drug design ensures the drug's Pharmacokinetic parameters and
particular compounds towards the precise target. Based on the network data
designed, EGCG were docked against PDB: 1N3U, using Dimethyl fumarate as the
standard reference. Such desirable quality of modified EGCG will create a spark
in the novel drug discovery of a bioenhancer. This slight evidence will support
a higher quantum of drug discovery in semisynthetic chemistry toward Metabolic
Complications.
References:
[2].
Tiwari S, Ndisang JF, 2014, The heme oxygenase system and
type-1 diabetes, Current pharmaceutical design, 20(9):1328-37. Epub 2013/08/28.
doi: 10.2174/13816128113199990552. PubMed PMID: 23978102.
[3].
Roberts MS, Burbelo PD, Egli-Spichtig D, Perwad F, Romero
CJ, Ichikawa S, et al., 2018, Autoimmune hyperphosphatemic tumoral calcinosis
in a patient with FGF23 autoantibodies, The Journal of clinical investigation, 128(12):5368-73.
Epub 2018/09/19. doi: 10.1172/jci122004. PubMed PMID: 30226830; PubMed Central
PMCID: PMCPMC6264742.
[4].
Pozzilli P, Pieralice S., 2018, Latent Autoimmune
Diabetes in Adults: Current Status and New Horizons, Endocrinology and
metabolism, 33(2):147-59. Epub 2018/06/28. doi: 10.3803/EnM.2018.33.2.147.
PubMed PMID: 29947172; PubMed Central PMCID: PMCPMC6021307.
[5].
Paschou SA, Papadopoulou-Marketou N, Chrousos GP,
Kanaka-Gantenbein C., 2018, On type 1 diabetes mellitus pathogenesis, Endocrine
connections,7(1):R38-r46. Epub 2017/12/02. doi: 10.1530/ec-17-0347. PubMed
PMID: 29191919; PubMed Central PMCID: PMCPMC5776665.
[6].
Rosenblum MD, Remedios KA, Abbas A K., 2015, Mechanisms
of human autoimmunity, The Journal of clinical investigation, 125(6):2228-33.
Epub 2015/04/22. doi: 10.1172/jci78088. PubMed PMID: 25893595; PubMed Central
PMCID: PMCPMC4518692.
[7].
Atkinson MA, Maclaren NK., 1994, The pathogenesis of
insulin-dependent diabetes mellitus, The New England journal of medicine, 331(21):1428-36.
Epub 1994/11/24. doi: 10.1056/nejm199411243312107. PubMed PMID: 7969282.
[8].
Eizirik DL, Colli ML, Ortis F., 2009, The role of
inflammation in insulitis and beta-cell loss in type 1 diabetes, Nature reviews Endocrinology, 5(4):219-26.
Epub 2009/04/09. doi: 10.1038/nrendo.2009.21. PubMed PMID: 19352320.
[9].
Martin SJ, Green DR, 1995, Protease activation during
apoptosis: Death by a thousand cuts?, Cell, 82(3):349-52. doi: https://doi.org/10.1016/0092-8674(95)90422-0.
[10]. Tomita T, 2017, Apoptosis
of pancreatic β-cells in Type 1 diabetes, Bosnian journal of basic medical sciences,
17(3):183-93. Epub 2017/04/04. doi: 10.17305/bjbms.2017.1961. PubMed PMID:
28368239; PubMed Central PMCID: PMCPMC5581966.
[11]. Galicia-Garcia U,
Benito-Vicente A, Jebari S, Larrea-Sebal A, Siddiqi H, Uribe KB, et al,. 2020,
Pathophysiology of Type 2 Diabetes Mellitus, International journal of molecular
sciences, 21(17). Epub 2020/09/03. doi: 10.3390/ijms21176275. PubMed PMID:
32872570; PubMed Central PMCID: PMCPMC7503727.
[12]. Riddle MC, Cefalu WT,
Evans PH, Gerstein HC, Nauck MA, Oh WK, et al., 2021, Consensus Report:
Definition and Interpretation of Remission in Type 2 Diabetes, The Journal of
Clinical Endocrinology & Metabolism, 107(1):1-9. doi:
10.1210/clinem/dgab585 %J The Journal of Clinical Endocrinology &
Metabolism.
[13]. Shoelson SE, Lee J,
Goldfine AB., 2006, Inflammation and insulin resistance, The Journal of
clinical investigation, 116(7):1793-801. Epub 2006/07/11. doi:
10.1172/jci29069. PubMed PMID: 16823477; PubMed Central PMCID: PMCPMC1483173.
[14]. Takaoka M, Nagata D,
Kihara S, Shimomura I, Kimura Y, Tabata Y, et al., 2009, .Periadventitial
adipose tissue plays a critical role in vascular remodeling, Circulation
research, 105(9):906-11. Epub 2009/09/19. doi: 10.1161/circresaha.109.199653.
PubMed PMID: 19762682.
[15]. Maliszewska K,
Kretowski A., 2021, Brown Adipose Tissue and Its Role in Insulin and Glucose
Homeostasis, Int J Mol Sci, 22(4):1530. PubMed PMID: doi:10.3390/ijms22041530.
[16]. Mileti E, Kwok KHM,
Andersson DP, Mathelier A, Raman A, Bäckdahl J, et al., 2021, Human White
Adipose Tissue Displays Selective Insulin Resistance in the Obese State,
Diabetes , 70(7):1486-97. doi: 10.2337/db21-0001 %J Diabetes.
[17]. Tomita T, 2016,
Apoptosis in pancreatic β-islet cells in Type 2 diabetes, Bosnian journal of
basic medical sciences, 16(3):162-79. Epub 2016/05/23. doi:
10.17305/bjbms.2016.919. PubMed PMID: 27209071; PubMed Central PMCID:
PMCPMC4978108.
[18]. Mitsuishi Y,
Motohashi H, Yamamoto M, 2012, The Keap1-Nrf2 system in cancers: stress
response and anabolic metabolism, Frontiers in oncology, 2:200. Epub
2012/12/29. doi: 10.3389/fonc.2012.00200. PubMed PMID: 23272301; PubMed Central
PMCID: PMCPMC3530133.
[19]. Kobayashi M, Yamamoto
M, 2006, Nrf2-Keap1 regulation of cellular defense mechanisms against
electrophiles and reactive oxygen species, Advances in enzyme regulation,
46:113-40. Epub 2006/08/05. doi: 10.1016/j.advenzreg.2006.01.007. PubMed PMID:
16887173.
[20]. Kansanen E, Kuosmanen
SM, Leinonen H, Levonen A L, 2013, The Keap1-Nrf2 pathwy: Mechanisms of
activation and dysregulation in cancer, Redox biology, 1(1):45-9. Epub
2013/09/12. doi: 10.1016/j.redox.2012.10.001. PubMed PMID: 24024136; PubMed
Central PMCID: PMCPMC3757665.
[21]. Canning P, Sorrell
FJ, Bullock AN, 2015, Structural basis of Keap1 interactions with Nrf2, Free
radical biology & medicine, 88(Pt B):101-7. Epub 2015/06/10. doi:
10.1016/j.freeradbiomed.2015.05.034. PubMed PMID: 26057936; PubMed Central
PMCID: PMCPMC4668279.
[22]. Tebay LE, Robertson
H, Durant ST, Vitale SR, Penning TM, Dinkova-Kostova AT, et al., 2015,
Mechanisms of activation of the transcription factor Nrf2 by redox stressors,
nutrient cues, and energy status and the pathways through which it attenuates
degenerative disease, Free radical biology & medicine, 88(Pt B):108-46.
Epub 2015/07/01. doi: 10.1016/j.freeradbiomed.06.021. PubMed PMID: 26122708;
PubMed Central PMCID: PMCPMC4659505.
[23]. Loboda A, Damulewicz
M, Pyza E, Jozkowicz A, Dulak J., 2016, Role of Nrf2/HO-1 system in
development, oxidative stress response and diseases: an evolutionarily
conserved mechanism, Cellular and Molecular Life Sciences, 73(17):3221-47. doi:
10.1007/s00018-016-2223-0.
[24]. Araujo J, Zhang M,
Yin F, 2012, Heme Oxygenase-1, Oxidation, Inflammation, and Atherosclerosis, 3(01):01-14.
doi: 10.3389/fphar.2012.00119.
[25]. Barañano DE, Ferris
CD, Snyder SH, 2021, Atypical neural messengers, Trends in Neurosciences,
24(2):99-106. doi: https://doi.org/10.1016/S0166-2236(00)01716-1.
[26]. Rochette L, Zeller M,
Cottin Y, Vergely C, 2018, Redox Functions of Heme Oxygenase-1 and Biliverdin
Reductase in Diabetes, Trends in Endocrinology & Metabolism,29(2):74-85.
doi: https://doi.org/10.1016/j.tem.2017.11.005.
[27]. Sedlak TW, Saleh M,
Higginson DS, Paul BD, Juluri KR, Snyder SH, 2009, Bilirubin and glutathione
have complementary antioxidant and cytoprotective roles, Proc Natl Acad Sci U S A, 106(13):5171-6. doi:
doi:10.1073/pnas.0813132106.
[28]. Seyoum A, Asres K,
El-Fiky FK, 2006, Structure-radical scavenging activity relationships of
flavonoids. Phytochemistry, 67(18):2058-70. Epub 2006/08/22. doi:
10.1016/j.phytochem.2006.07.002. PubMed PMID: 16919302.
[29]. Ru J, Li P, Wang J,
Zhou W, Li B, Huang C, et al., 2014, TCMSP: a database of systems pharmacology
for drug discovery from herbal medicines, Journal of Cheminformatics, 6(1):13.
doi: 10.1186/1758-2946-6-13.
[30]. Ouassou H, Zahidi T,
Bouknana S, Bouhrim M, Mekhfi H, Ziyyat A, et al., 2018, Inhibition of
α-Glucosidase, Intestinal Glucose Absorption, and Antidiabetic Properties by
Caralluma europaea, Evidence-based complementary and alternative medicine: eCAM,
9589472. Epub 2018/09/20. doi: 10.1155/2018/9589472. PubMed PMID: 30228829;
PubMed Central PMCID: PMCPMC6136516.
[31]. Turner N, Zeng X-Y,
Osborne B, Rogers S, Ye J-M, 2016, Repurposing Drugs to Target the Diabetes
Epidemi, Trends in Pharmacological Sciences. 37(5):379-89. doi: https://doi.org/10.1016/j.tips.2016.01.007.
[32]. Wang B, Sun J, Li X,
Zhou Q, Bai J, Shi Y, et al., 2013, Resveratrol prevents suppression of
regulatory T-cell production, oxidative stress, and inflammation of mice prone
or resistant to high-fat diet–induced obesity, Nutrition
Research.33(11):971-81. doi: https://doi.org/10.1016/j.nutres.2013.07.016.
[33]. Song M-Y, Kim E-K,
Moon W-S, Park J-W, Kim H-J, So H-S, et al., 2009, Sulforaphane protects
against cytokine- and streptozotocin-induced β-cell damage by suppressing the
NF-κB pathway. Toxicology and Applied Pharmacology. 235(1):57-67. doi: https://doi.org/10.1016/j.taap.2008.11.007.
[34]. Rashid K, Sil PC,
2015, Curcumin enhances recovery of pancreatic islets from cellular stress
induced inflammation and apoptosis in diabetic rats, Toxicology and Applied
Pharmacology, 282(3):297-310. doi: https://doi.org/10.1016/j.taap.2014.12.003.
[35]. Coskun O, Kanter M,
Korkmaz A, Oter S, 2005, Quercetin, a flavonoid antioxidant, prevents and
protects streptozotocin-induced oxidative stress and β-cell damage in rat
pancreas. Pharmacological Research, 51(2):117-23. doi: https://doi.org/10.1016/j.phrs.2004.06.002.
[36]. Chen C-Y, Jang J-H,
Li M-H, Surh Y-J, 2005, Resveratrol upregulates heme oxygenase-1 expression via
activation of NF-E2-related factor 2 in PC12 cells, Biochemical and Biophysical
Research Communications, 2005;331 (4):993-1000. doi: https://doi.org/10.1016/j.bbrc.2005.03.237.
[37]. Lin C-F, Chueh T-H,
Chung C-H, Chung S-D, Chang T-C, Chien C-T, 2020., Sulforaphane improves
voiding function via the preserving mitochondrial function in diabetic rats,
Journal of the Formosan Medical Association, 119(9):1422-30. doi: https://doi.org/10.1016/j.jfma.2019.11.017.
[38]. Nicolai A, Li M, Kim
DH, Peterson SJ, Vanella L, Positano V, et al., 2009, Heme Oxygenase-1
Induction Remodels Adipose Tissue and Improves Insulin Sensitivity in Obesity-Induced
Diabetic Rats, Hypertenssion, 53(3):508-15. doi:
doi:10.1161/HYPERTENSIONAHA.108.124701.
[39]. Lim D-W, Kim H, Kim
Y-M, Chin Y-W, Park W-H, Kim J-E, 2019, Drug repurposing in alternative
medicine: herbal digestive Sochehwan exerts multifaceted effects against
metabolic syndrome, Scientific Reports, 9(1):9055. doi:
10.1038/s41598-019-45099-x.
[40]. Pushpakom S, Iorio F,
Eyers PA, Escott KJ, Hopper S, Wells A, et al., 2019, Drug repurposing:
progress, challenges and recommendations, Nature Reviews Drug Discovery,
18(1):41-58. doi: 10.1038/nrd.2018.168.
[41]. Gonzalez-Alfonso JL,
Peñalver P, Ballesteros AO, Morales JC, Plou FJ, 2019, Effect of
α-Glucosylation on the Stability, Antioxidant Properties, Toxicity, and
Neuroprotective Activity of (–)-Epigallocatechin Gallate, 6(1):1-10. doi:
10.3389/fnut.2019.00030.
[42]. Hong Z, Xu Y, Yin
J-F, Jin J, Jiang Y, Du Q, 2014, Improving the Effectiveness of
(−)-Epigallocatechin Gallate (EGCG) against Rabbit Atherosclerosis by
EGCG-Loaded Nanoparticles Prepared from Chitosan and Polyaspartic Acid, Journal
of Agricultural and Food Chemistry, 62(52):12603-9. doi: 10.1021/jf504603n.
[43]. Sharifi-Rad M,
Pezzani R, Redaelli M, Zorzan M, Imran M, Ahmed Khalil A, et al., 2020,
Preclinical Activities of Epigallocatechin Gallate in Signaling Pathways in
Cancer, Molecules, 25(3):467. PubMed PMID: doi:10.3390/molecules25030467.
[44]. Wu CC, Hsu MC, Hsieh
CW, Lin JB, Lai PH, Wung BS, 2006, Upregulation of heme oxygenase-1 by
Epigallocatechin-3-gallate via the phosphatidylinositol 3-kinase/Akt and ERK
pathways, Life Sciences, 78(25):2889-97. doi: https://doi.org/10.1016/j.lfs.2005.11.013.
[45]. Zhou T, Zhu M, Liang
Z, 2018, (-)-Epigallocatechin-3-gallate modulates peripheral immunity in the
MPTP-induced mouse model of Parkinson's disease, Mol Med Rep, 17(4):4883-8.
doi: 10.3892/mmr.2018.8470.
[46]. Irfan,
M, Lee, YY, Lee, KJ, Kim, SD, & Rhee, MH, 2022, Comparative antiplatelet
and antithrombotic effects of red ginseng and fermented red ginseng extracts, Journal
of Ginseng Research, 46(3), 387-395.
[47]. Singla,
N, Gupta, G, Kulshrestha, R, Sharma, K, Bhat, AA, Mishra, R, & Gupta, S,
2024, Daidzein in Traditional Chinese Medicine: A Deep Dive into Its
Ethnomedicinal and Therapeutic Applications, Pharmacological
Research-Modern Chinese Medicine, 100460.
[48]. Wang,
J., Behl, T., Rana, T., Sehgal, A., Wal, P., Saxena, B., & Singla, R. K.,
2024, Exploring the Pathophysiological Influence of Heme Oxygenase-1 on
Neuroinflammation and Depression: A Study of Phytotherapeutic-Based Modulation, Phytomedicine,
155466.