Understand the Fatty Acid Metabolic Reprogramming of Immune Cells in Colorectal Cancer
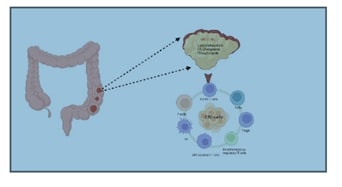
Abstract:
Colorectal cancer (CRC) is a prevalent and
challenging type of cancer worldwide. Recent research has focused intensively
on understanding the molecular and pathological mechanisms of CRC because of
the poor prognosis associated with its treatment. The tumor microenvironment
(TME) is crucial in tumor progression and has been the subject of extensive
investigation. Metabolic reprogramming has become a central focus in cancer
research, with numerous studies emphasizing its importance in CRC.
Specifically, the reprogramming of fatty acids has been found to alter the
energy and nutrient composition within the tumor microenvironment, affecting
the complex interaction between immune cells, especially macrophages and T
cells, and associated immune factors. This disruption can influence the tumor's
ability to evade immune surveillance. Our in-depth analysis highlights the role
of lipid metabolism processes in shaping the immune microenvironment of
colorectal cancer tumors, revealing the regulatory impact of fatty acid
metabolism on CRC development. The potential impact of this research on
improving CRC treatment is significant, underscoring the importance of
contribution to this field.
References:
[1].
Chen,
X., Ma, Z., Yi, Z., Wu, E., Shang, Z., Tuo, B., and Liu, X., 2024, The Effects
of Metabolism on the Immune Microenvironment in Colorectal Cancer, Cell Death
Discovery, 10(1), 118. https://doi.org/10.1038/s41420-024-01865-z
[2].
Marcellinaro,
R., Spoletini, D., Grieco, M., Avella, P., Cappuccio, M., Troiano, R., and
Carlini, M., 2023, Colorectal Cancer: Current Updates and Future Perspectives. Journal
of Clinical Medicine, 13(1), 40. https://doi.org/10.3390/jcm13010040
[3].
Chung,
R. Y. N., Tsoi, K. K., Kyaw, M. H., Lui, A. R., Lai, F. T., and Sung, J. J. Y.,
2019, A Population-Based Age-Period-Cohort Study of Colorectal Cancer Incidence
Comparing Asia Against the West. Cancer Epidemiology, 59, 29-36. https://doi.org/10.1016/j.canep.2019.01.007
[4].
Zhang,
S., Lv, K., Liu, Z., Zhao, R., and Li, F.,2024, Fatty Acid Metabolism of Immune
Cells: A New Target of Tumour Immunotherapy. Cell Death Discovery,
10(1), 39. https://doi.org/10.1038/s41420-024-01807-9
[5].
Liberti,
M. V., and Locasale, J. W., 2016, The Warburg Effect: How Does it Benefit
Cancer Cells? Trends in Biochemical Sciences, 41(3), 211-218. https://doi.org/10.1016/j.tibs.2015.12.001
[6].
Akter,
R., Awais, M., Boopathi, V., Ahn, J. C., Yang, D. C., Kang, S. C., and Jung, S.
K., 2024, Inversion of the Warburg Effect: Unraveling the Metabolic Nexus
between Obesity and Cancer. ACS Pharmacology & Translational Science, 7(3),
560-569. https://doi.org/10.1021/acsptsci.3c00301
[7].
Iranpanah
,A., Majnooni, M. B., Biganeh, H., Amirian, R., Rastegari-Pouyani, M., Filosa,
R., Cheang, W. S, Fakhri, S., and Khan, H., 2024, Exploiting New Strategies in
Combating Head and Neck Carcinoma: A Comprehensive Review on Phytochemical
Approaches Passing Through PI3K/Akt/mTOR Signaling Pathway. Phytother Res,
Jul;38(7):3736-3762. https://doi.org/10.1002/ptr.8228
[8].
Koundouros,
N., and Poulogiannis, G., 2020, Reprogramming of Fatty Acid Metabolism in
Cancer. British Journal of Cancer, 122(1), 4-22. https://doi.org/10.1038/s41416-019-0650-z
[9].
Shao,
N., Qiu, H., Liu, J., Xiao, D., Zhao, J., Chen, C., and Xu, L., 2024, Targeting
Lipid Metabolism of Macrophages: A New Strategy for Tumor Therapy. Journal
of Advanced Research, S2090-1232. https://doi.org/10.1016/j.jare.2024.02.009
[10].
Wu,
Y., Pu, X., Wang, X., and Xu, M., 2024, Reprogramming of Lipid Metabolism in
the Tumor Microenvironment: A Strategy for Tumor Immunotherapy. Lipids in
Health and Disease, 23(1), 35. https://doi.org/10.1186/s12944-024-02024-0
[11].
Chen,
X., Chen, S., and Yu, D., 2020, Metabolic Reprogramming of Chemoresistant
Cancer Cells and the Potential Significance of Metabolic Regulation in the
Reversal of Cancer Chemoresistance. Metabolites, 10(7), 289. https://doi.org/10.3390/metabo10070289
[12].
Luo,
Y., Wang, H., Liu, B., and Wei, J., 2022, Fatty Acid Metabolism and Cancer
Immunotherapy. Current Oncology Reports, 24(5), 659-670. https://doi.org/10.1007/s11912-022-01223-1
[13].
Liang,
X. H., Chen, X. Y., Yan, Y., Cheng, A. Y., Lin, J. Y., Jiang, Y. X., and Luan,
X., 2024, Targeting Metabolism to Enhance Immunotherapy Within Tumor
Microenvironment. Acta Pharmacologica Sinica, 1-12. https://doi.org/10.1038/s41401-024-01304-w
[14].
Jin,
J., Byun, J. K., Choi, Y. K., and Park, K. G., 2023, Targeting Glutamine
Metabolism as a Therapeutic Strategy for Cancer. Experimental &
Molecular Medicine, 55(4), 706-715. https://doi.org/10.1038/s12276-023-00971-9
[15].
Pascual,
G., Majem, B., and Benitah, S. A., 2024, Targeting Lipid Metabolism in Cancer
Metastasis. Biochimica et Biophysica Acta (BBA)-Reviews on Cancer, 1879(1),
189051. https://doi.org/10.1016/j.bbcan.2023.189051
[16].
Wang,
R., Yan, Q., Liu, X., and Wu, J., 2024. Unraveling Lipid Metabolism
Reprogramming for Overcoming Drug Resistance in Melanoma. Biochemical
Pharmacology, 116122. https://doi.org/10.1016/j.bcp.2024.116122
[17].
Gupta,
A., Das, D., and Taneja, R., 2024, Targeting Dysregulated Lipid Metabolism in
Cancer with Pharmacological Inhibitors. Cancers, 16(7), 1313. https://doi.org/10.3390/cancers16071313
[18].
Krauß,
D., Fari, O., and Sibilia, M., 2022, Lipid Metabolism Interplay in CRC—An
Update. Metabolites, 12(3), 213. https://doi.org/10.3390/metabo12030213
[19].
Endo,
Y., Kanno, T., and Nakajima, T., 2022, Fatty Acid Metabolism in T-cell Function
and Differentiation. International Immunology, 34(11), 579-587. https://doi.org/10.1093/intimm/dxac025
[20].
Liu,
X. F., Shao, J. H., Liao, Y. T., Wang, L. N., Jia, Y., Dong, P. J., and Zhang,
X., 2023, Regulation of Short-Chain Fatty Acids in the Immune System. Frontiers
in Immunology, 14, 1186892. https://doi.org/10.3389/fimmu.2023.1186892
[21].
Kim,
J. H., Kim, B. S., and Lee, S. K., 2020, Regulatory T Cells in Tumor
Microenvironment and Approach for Anticancer Immunotherapy. Immune Network,
20(1). https://doi.org/10.4110/in.2020.20.e4
[22].
Zhang,
Y., Kurupati, R., Liu, L., Zhou, X. Y., Zhang, G., Hudaihed, A., and Ertl, H.
C., 2017, Enhancing CD8+ T Cell Fatty Acid Catabolism Within a Metabolically
Challenging Tumor Microenvironment Increases the Efficacy of Melanoma
Immunotherapy. Cancer cell, 32(3), 377-391. https://doi.org/10.1016/j.ccell.2017.08.004
[23].
Sun,
P., Zhang, X., Wang, R. J., Ma, Q. Y., Xu, L., Wang, Y., and Meng, L. H., 2021,
PI3Kα Inhibitor CYH33 Triggers Antitumor Immunity in Murine Breast Cancer by
Activating CD8+ T Cells and Promoting Fatty Acid Metabolism. Journal for Immunotherapy
of Cancer, 9(8). https://doi.org/10.1136/jitc-2021-003093
[24].
Wang,
Z., Little, N., Chen, J., Lambesis, K. T., Le, K. T., Han, W., and Lu, J., 2021,
Immunogenic Camptothesome Nanovesicles Comprising Sphingomyelin-Derived
Camptothecin Bilayers for Safe and Synergistic Cancer Immunochemotherapy.
Nature Nanotechnology, 16(10), 1130-1140. https://doi.org/10.1038/s41565-021-00950-z
[25].
Wu,
H., Han, Y., Rodriguez Sillke, Y., Deng, H., Siddiqui, S., Treese, C., and Glauben,
R., 2019, Lipid Droplet‐Dependent Fatty Acid Metabolism Controls the Immune
Suppressive Phenotype of Tumor‐Associated Macrophages. EMBO Molecular
Medicine, 11(11), e10698. https://doi.org/10.15252/emmm.201910698
[26].
Düvel,
K., Yecies, J. L., Menon, S., Raman, P., Lipovsky, A. I., Souza, A. L., and Manning,
B. D., 2010, Activation of a Metabolic Gene Regulatory Network Downstream of
mTOR Complex 1. Molecular cell, 39(2), 171-183. https://doi.org/10.1016/j.molcel.2010.06.022
[27].
Ma,
X., Bi, E., Lu, Y., Su, P., Huang, C., Liu, L., and Yi, Q., 2019, Cholesterol Induces
CD8+ T Cell Exhaustion in the Tumor Microenvironment. Cell Metabolism, 30(1),
143-156. https://doi.org/10.1016/j.cmet.2019.04.002
[28].
Ventura,
A., Kirsch, D. G., McLaughlin, M. E., Tuveson, D. A., Grimm, J., Lintault, L., and
Jacks, T., 2007, Restoration of p53 Function Leads to Tumour Regression in Vivo.
Nature, 445(7128), 661-665. https://doi.org/10.1038/nature05541
[29].
Keerthivasan,
S., Aghajani, K., Dose, M., Molinero, L., Khan, M. W., Venkateswaran, V., and
Gounari, F., 2014, β-Catenin Promotes Colitis and Colon Cancer Through
Imprinting of Proinflammatory Properties in T Cells. Science Translational
Medicine, 6(225), 225ra28-225ra28. https://doi.org/10.1126/scitranslmed.3007607
[30].
Yu,
F. X., Zhao, B., Panupinthu, N., Jewell, J. L., Lian, I., Wang, L. H., and Guan,
K. L., 2012, Regulation of the Hippo-YAP Pathway by G-Protein-Coupled Receptor
Signaling. Cell, 150(4), 780-791. https://doi.org/10.1016/j.cell.2012.06.037
[31].
Zhang,
D., Shi, R., Xiang, W., Kang, X., Tang, B., Li, C., and Miao, H., 2020, The
Agpat4/LPA Axis In Colorectal Cancer Cells Regulates Antitumor Responses Via
p38/p65 Signaling in Macrophages. Signal Transduction and Targeted Therapy,
5(1), 24. https://doi.org/10.1038/s41392-020-0117-y
[32].
Chen,
J., Zhu, H., Yin, Y., Jia, S., and Luo, X., 2022, Colorectal Cancer: Metabolic
Interactions Reshape the Tumor Microenvironment. Biochimica et Biophysica Acta
(BBA)-Reviews on Cancer, 1877(5), 188797. https://doi.org/10.1016/j.bbcan.2022.188797
[33].
Wang,
Z., Cordova, L. E., Chalasani, P., and Lu, J.,2022, Camptothesome Potentiates
PD-L1 Immune Checkpoint Blockade for Improved Metastatic Triple-Negative Breast
Cancer Immunochemotherapy. Molecular Pharmaceutics, 19(12), 4665-4674. https://doi.org/10.1016/j.bbcan.2022.188797