Green Synthesis and Characterization of Cobalt Nanoparticles Using Butea Monosperma Flower Extract and their Biocompatibility Studies
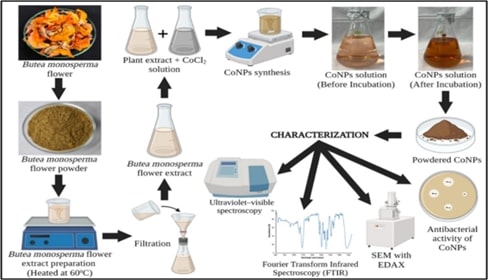
Abstract:
This
study explores the green synthesis of cobalt nanoparticles (CoNPs) using Butea
monosperma flower extract, highlighting their antibacterial efficacy, toxicity,
and biocompatibility. Utilizing a plant-based reduction method, cobalt ions
were reduced and stabilized by the bioactive compounds in the flower extract,
forming CoNPs with notable uniformity and stability. Characterization
techniques, including, UV-VIS spectroscopy, Fourier-transform infrared (FTIR)
spectroscopy, and scanning electron microscopy (SEM) with energy-dispersive
X-ray spectroscopy (EDAX), confirmed the formation and nature of the
nanoparticles, which exhibited an average size of 10-20 nm. The synthesized
CoNPs demonstrated significant antibacterial activity against both Gram-positive
and Gram-negative bacteria, suggesting their potential as effective
antimicrobial agents. Toxicity assessment using zebrafish, and brine shrimp
lethality assay (BSLA), revealed that the nanoparticles exhibited minimal
toxicity. Biocompatibility studies further
indicated that CoNPs had no adverse effects on cellular morphology or
proliferation, highlighting their suitability for biomedical applications. The
eco-friendly synthesis method not only provides a sustainable approach to
nanoparticle production but also enhances the potential for their safe application
in medical and environmental fields. The findings underscore the promise of B.
monosperma-mediated CoNPs as a viable alternative to chemically synthesized
nanoparticles, with significant implications for antibacterial therapies and
biocompatible materials.
References:
[1].
Vodyashkin, A. A.,
Kezimana, P., Prokonov, F. Y., Vasilenko, I. A., and Stanishevskiy, Y. M.,
2022. Current Methods for Synthesis and Potential Applications of Cobalt
Nanoparticles: A review. Crystals, 12(2),272. https://doi.org/10.3390/cryst12020272
[2].
Alsaiari, N. S., Alzahrani, F. M., Amari, A.,
Osman, H., Harharah, H. N., Elboughdiri, N., and Tahoon, M. A., 2023. Plant and
Microbial Approaches as Green Methods for the Synthesis of Nanomaterials:
Synthesis, Applications, and Future Perspectives. Molecules, 28(1), 463.
https://doi.org/10.3390/molecules28010463
[3].
Srivathsan, J., Sivakami, V., Ramachandran,
B., Harikrishna, K.S., Vetriselvi, S. and Kumar, D.J.M., 2012. Synthesis of
silver nanoparticles and its effect on soil bacteria. J. Microbiol.
Biotech. Res, 2(6), pp.871-874.
[4].
Khusnuriyalova, A. F., Caporali, M.,
Hey‐Hawkins, E., Sinyashin, O. G., and Yakhvarov, D. G., 2021. Preparation of Cobalt
Nanoparticles. European Journal of Inorganic Chemistry, 2021(30),
3023-3047. https://doi.org/10.1002/ejic.202100367
[5].
Pusta, A., Tertis, M., Crăciunescu, I., Turcu,
R., Mirel, S., and Cristea, C., 2023. Recent Advances in the Development of
Drug Delivery Applications of Magnetic Nanomaterials. Pharmaceutics,
15(7), 1872. https://doi.org/10.3390/pharmaceutics15071872
[6].
Farzin, A., Etesami, S. A., Quint, J., Memic,
A., and Tamayol, A., 2020. Magnetic Nanoparticles in Cancer Therapy and
Diagnosis. Advanced Healthcare Materials, 9(9), 1901058. https://doi.org/10.1002/adhm.201901058
[7].
Ryntathiang, I., Behera, A., Richard, T., and
Jothinathan, M. K. D., 2024. An Assessment of the in Vitro Antioxidant Activity
of Cobalt Nanoparticles Synthesized from Millettia Pinnata, Butea Monosperma,
and Madhuca Indica Extracts: A Comparative Study. Cureus, 16(4), e59112.
https://doi.org/10.7759/cureus.59112
[8].
Abass, A. A., Alaarage, W. K., Abdulrudha, N. H.,
and Haider, J., 2021. Evaluating the Antibacterial Effect of Cobalt
Nanoparticles Against Multi-Drug Resistant Pathogens. Journal of Medicine
and Life, 14(6), 823. https://doi.org/10.25122/jml-2021-0270
[9].
Kharade Suvarta, D., Nikam Gurunath, H., Mane
Gavade Shubhangi, J., Patil Sachinkumar, R., and Gaikwad Kishor, V., 2020.
Biogenic Synthesis of Cobalt Nanoparticles using Hibiscus Cannabinus Leaf
Extract and their Antibacterial Activity. Research Journal of Chemistry and
Environment, 24(5), 9-13.
https://www.researchgate.net/profile/Sachinkumar-Patil/publication/340844918
[10].
Cruz, J. C., Nascimento, M. A., Amaral, H. A., Lima, D. S., Teixeira, A.
P. C., and Lopes, R. P., 2019. Synthesis and Characterization
of Cobalt Nanoparticles for Application in the Removal of Textile Dye. Journal
of Environmental Management, 242, 220-228. https://doi.org/10.1016/j.jenvman.2019.04.059
[11].
Fardood, S. T., Forootan, R., Moradnia, F.,
Afshari, Z., and Ramazani, A., 2020. Green Synthesis, Characterization, and
Photocatalytic Activity of Cobalt Chromite Spinel Nanoparticles. Materials
Research Express, 7(1), p.015086. https://doi.org/10.1088/2053-1591/ab6c8d
[12].
Kus-Liśkiewicz, M., Fickers, P., and Ben
Tahar, I., 2021. Biocompatibility and Cytotoxicity of Gold Nanoparticles:
Recent Advances in Methodologies and Regulations. International Journal of
Molecular Sciences, 22(20), 10952. https://doi.org/10.3390/ijms222010952
[13].
Ansari, S. M., Bhor, R. D., Pai, K. R., Sen,
D., Mazumder, S., Ghosh, K., Kolekar, Y. D., and Ramana, C.V., 2017. Cobalt Nanoparticles
for Biomedical Applications: Facile Synthesis, Physiochemical Characterization,
Cytotoxicity Behavior and Biocompatibility. Applied Surface Science,
414, 171-187. https://doi.org/10.1016/j.apsusc.2017.03.002
[14].
Bittner, M., Štern, A., Smutná, M.,
Hilscherová, K., and Žegura, B., 2021. Cytotoxic and Genotoxic Effects of
Cyanobacterial and Algal Extracts—Microcystin and Retinoic Acid Content. Toxins,
13(2), 107. https://doi.org/10.3390/toxins13020107
[15].
Zhao, W., Chen, Y., Hu, N., Long, D., and Cao,
Y., 2024. The Uses of Zebrafish (Danio rerio) as an in Vivo Model for
Toxicological Studies: A Review Based on Bibliometrics. Ecotoxicology and
Environmental Safety, 272, 116023. https://doi.org/10.1016/j.ecoenv.2024.116023
[16].
Modarresi Chahardehi, A., Arsad, H., and Lim,
V., 2020. Zebrafish as a Successful Animal Model for Screening Toxicity of
Medicinal Plants. Plants, 9(10),1345. https://doi.org/10.3390/plants9101345
[17].
Haque, E., and Ward, A. C., 2018. Zebrafish as
a Model to Evaluate Nanoparticle Toxicity. Nanomaterials, 8(7), 561.
https://doi.org/10.3390/nano8070561
[18].
Miyawaki, I., 2020. Application of Zebrafish
to Safety Evaluation in Drug Discovery. Journal of Toxicologic Pathology,
33(4), 197-210. https://doi.org/10.1293/tox.2020-0021
[19].
Payne-Sturges, D. C., Scammell, M. K., Levy,
J. I., Cory-Slechta, D. A., Symanski, E., Carr Shmool, J. L., Laumbach, R.,
Linder, S., and Clougherty, J. E., 2018. Methods for Evaluating the Combined
Effects of Chemical and Nonchemical Exposures for Cumulative Environmental
Health Risk Assessment. International Journal of Environmental Research and
Public Health, 15(12), 2797. https://doi.org/10.3390/ijerph15122797
[20].
Cassar, S., Adatto, I., Freeman, J. L., Gamse,
J. T., Iturria, I., Lawrence, C., Muriana, A., Peterson, R. T., Van Cruchten,
S., and Zon, L. I., 2019. Use of Zebrafish in Drug Discovery Toxicology. Chemical
Research in Toxicology, 33(1), 95-118. https://doi.org/10.1021/acs.chemrestox.9b00335
[21].
Frisch, E., Clavier, L., Belhamdi, A., Vrana,
N. E., Lavalle, P., Frisch, B., Heurtault, B., and Gribova, V., 2023.
Preclinical in Vitro Evaluation of Implantable Materials: Conventional
Approaches, New Models and Future Directions. Frontiers in Bioengineering
and Biotechnology, 11, 1193204. https://doi.org/10.3389/fbioe.2023.1193204
[22].
Othman, Z., Pastor, B. C., van Rijt, S., and
Habibovic, P., 2018. Understanding Interactions Between Biomaterials and
Biological Systems Using Proteomics. Biomaterials, 167, 191-204. https://doi.org/10.1016/j.biomaterials.2018.03.020
[23].
Egbuna, C., Parmar, V. K., Jeevanandam, J.,
Ezzat, S. M., Patrick-Iwuanyanwu, K. C., Adetunji, C. O., Khan, J., Onyeike, E.
N., Uche, C. Z., Akram, M., and Ibrahim, M. S., 2021. Toxicity of Nanoparticles
in Biomedical Application: Nanotoxicology. Journal of Toxicology,
2021(1), 9954443. https://doi.org/10.1155/2021/9954443
[24].
Witika, B. A., Makoni, P. A., Matafwali, S. K.,
Chabalenge, B., Mwila, C., Kalungia, A. C., Nkanga, C. I., Bapolisi, A. M. and
Walker, R. B., 2020. Biocompatibility of Biomaterials for Nanoencapsulation:
Current Approaches. Nanomaterials, 10(9),1649. https://doi.org/10.3390/nano10091649
[26].
Munusamy, T., and Shanmugam, R., 2023. Green
Synthesis of Copper Oxide Nanoparticles Synthesized by Terminalia chebula Dried
Fruit Extract: Characterization and Antibacterial Action. Cureus,
15(12), e50142. https://doi.org/10.7759/cureus.50142
[27]. Anandan, J., and Shanmugam, R., 2024. Antioxidant, Anti-inflammatory,
and Antimicrobial Activity of the Kalanchoe Pinnata and Piper Longum
Formulation Against Oral Pathogens. Cureus, 16(4), e57824. https://doi.org/10.7759/cureus.57824
[28].
Rajeshkumar, S., Santhoshkumar, J., Vanaja,
M., Sivaperumal, P., Ponnanikajamideen, M., Ali, D., and Arunachalam, K., 2022.
Evaluation of Zebrafish Toxicology and Biomedical Potential of Aeromonas
Hydrophila Mediated Copper Sulfide Nanoparticles. Oxidative Medicine and
Cellular Longevity, 2022(1), 7969825. https://doi.org/10.1155/2022/7969825
[29]. Sankar, H. N., Shanmugam, R., and Anandan, J., 2024. Green Synthesis of
Euphorbia Tirucalli-Mediated Titanium Dioxide Nanoparticles Against Wound
Pathogens. Cureus, 16(2), e53939. https://doi.org/10.7759/cureus.53939
[30]. Ameena, M., Arumugham,
M., Ramalingam, K., Rajeshkumar, S., and Perumal, E., 2023. Cytocompatibility and Wound Healing Activity of Chitosan
Thiocolchicoside Lauric Acid Nanogel in Human Gingival Fibroblast Cells. Cureus, 15(8), e43727. https://doi.org/10.7759/cureus.43727
[31].
Ali, H., Dixit, S., and Alarifi, S., 2024. Biosynthesis
and Screening of Cobalt Nanoparticles Using Citrus Species for Antimicrobial
Activity. Open Chemistry, 22(1), 20240021. https://doi.org/10.1515/chem-2024-0021
[32].
Ali, S. G., Ansari, M. A., Alzohairy, M. A.,
Alomary, M. N., Jalal, M., AlYahya, S., Asiri, S. M. M. and Khan, H. M., 2020.
Effect of Biosynthesized ZnO Nanoparticles on Multi-Drug Resistant Pseudomonas Aeruginosa.
Antibiotics, 9(5), 260. https://doi.org/10.3390/antibiotics9050260
[33].
Kalanakoppal Venkatesh, Y., Mahadevaiah, R.,
Haraluru Shankaraiah, L., Ramappa, S., and Sannagoudar Basanagouda, A., 2018.
Preparation of a CaO Nanocatalyst and its Application for Biodiesel Production
Using Butea Monosperma Oil: An Optimization Study. Journal of the American
Oil Chemists' Society, 95(5), 635-649. https://doi.org/10.1002/aocs.12079
[34].
Kumar, V., Harini, R., Anitha, G., and
Nagaraju, G., 2024. Facile Green Synthesis of Zn Doped MoO3 Nanoparticles and
its Photocatalytic and Photoluminescence Studies. Journal of Molecular
Structure, 1312, 138494. https://doi.org/10.1016/j.molstruc.2024.138494
[35].
Mubraiz, N., Bano, A., Mahmood, T., and Khan,
N., 2021. Microbial and Plant Assisted Synthesis of Cobalt Oxide Nanoparticles
and their Antimicrobial Activities. Agronomy, 11(8), https://doi.org/1607.10.3390/agronomy11081607
[36].
Mmelesi, O. K., Masunga, N., Kuvarega, A.,
Nkambule, T.T., Mamba, B. B., and Kefeni, K. K., 2021. Cobalt Ferrite
Nanoparticles and Nanocomposites: Photocatalytic, Antimicrobial Activity and
Toxicity in Water Treatment. Materials Science in Semiconductor Processing,
123, 105523. https://doi.org/10.1016/j.mssp.2020.105523
[37].
Alyami, M. H., Fakhry, A. M., El Halfawy, N. M.,
Toto, S. M., Sedky, N. K., Yassin, H. A., Fahmy, S. A., and Mokhtar, F. A.,
2023. Retama Monosperma Chemical Profile, Green Synthesis of Silver
Nanoparticles, and Antimicrobial Potential: A Study Supported by Network
Pharmacology and Molecular Docking. RSC Advances, 13(37), 26213-26228. https://doi.org/10.1039/D3RA05116A
[38].
Zafar, N., Madni, A., Khalid, A., Khan, T.,
Kousar, R., Naz, S. S. and Wahid, F., 2020. Pharmaceutical and Biomedical
Applications of Green Synthesized Metal and Metal Oxide Nanoparticles. Current
Pharmaceutical Design, 26(45), 5844-5865. https://doi.org/10.2174/1381612826666201126144805
[39].
Siddiqi, K. S., Rahman, A., Tajuddin, N., and
Husen, A., 2018. Properties of Zinc Oxide Nanoparticles and their Activity
Against Microbes. Nanoscale Research Letters, 13, 1-13. https://doi.org/10.1186/s11671-018-2532-3
[40].
d’Amora, M., Schmidt, T. J. N., Konstantinidou, S., Raffa, V., De
Angelis, F., and Tantussi, F., 2022. Effects of Metal
Oxide Nanoparticles in Zebrafish. Oxidative Medicine and Cellular Longevity,
2022(1), 3313016. https://doi.org/10.1155/2022/3313016
[41].
Chen, J., Lei, L., Mo, W., Dong, H., Li, J.,
Bai, C., Huang, K., Truong, L., Tanguay, R. L., Dong, Q., and Huang, C., 2021.
Developmental Titanium Dioxide Nanoparticle Exposure Induces Oxidative Stress
and Neurobehavioral Changes in Zebrafish. Aquatic Toxicology, 240,
105990. https://doi.org/10.1016/j.aquatox.2021.105990
[42].
Yan, S., Qian, Y., Haghayegh, M., Xia, Y.,
Yang, S., Cao, R., and Zhu, M., 2024. Electrospun Organic/Inorganic Hybrid
Nanofibers for Accelerating Wound Healing: A Review. Journal of Materials
Chemistry B, 12, 3171-3190. https://doi.org/10.1039/D4TB00149D
[43].
Chakraborty, C., Sharma, A. R., Sharma, G.,
and Lee, S. S., 2016. Zebrafish: A Complete Animal Model to Enumerate the
Nanoparticle Toxicity. Journal of Nanobiotechnology, 14,1-13. https://doi.org/10.1186/s12951-016-0217-6