Molecular and Epigenetic Studies on the Effect of Hesperidin on IRS-1/Akt/GLUT4 Signaling Molecules in the Gastrocnemius Muscle of Streptozotocin-induced Type-2 Diabetic Rats
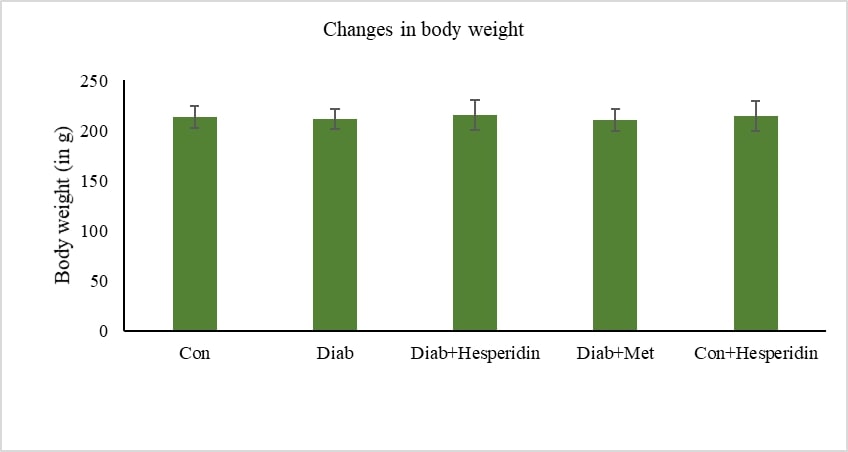
Abstract:
Diabetes mellitus is a significant global health issue, affecting 425
million people worldwide, with projections estimating an increase to 629
million by 2045. The need for potent pharmacological agents is urgent, as
current oral hypoglycemic drugs have adverse side effects. Hesperidin, a
bioflavonoid with anti-hyperglycemic and anti-hyperlipidemic properties, offers
promise as a natural therapeutic option. This study aimed to evaluate
hesperidin's molecular and epigenetic effects on insulin signal transduction in
the gastrocnemius muscle of STZ-induced type 2 diabetic rats. Methods involved
dividing fully-grown male Wistar rats into five groups: Healthy control,
STZ-induced diabetic, Diabetes+Hesperidin (100mg/kg), Diabetes+Metformin
(50mg/kg), and Control+Hesperidin. At the experiment's conclusion, blood
samples and gastrocnemius muscle tissues were collected to measure fasting
blood glucose, serum insulin, antioxidant enzymes, oxidative stress markers,
and histopathological and mRNA expression of insulin signalling molecules. Data
were analyzed using one-way ANOVA, with significance set at p<0.05. Results
indicated that STZ-induced diabetic rats exhibited significant increases in
hyperglycemia, hyperinsulinemia, dyslipidemia, and oxidative stress markers,
along with reduced superoxide dismutase (SOD) activity. Histopathological
analysis revealed reduced muscle fibres and disrupted skeletal fibres.
Additionally, mRNA expression of IRS-1, Akt, and GLUT 4 was significantly
reduced. Remarkably, hesperidin treatment normalized these altered parameters.
In conclusion, hesperidin effectively regulates insulin signalling in skeletal
muscle, reducing diabetic risk. Thus, hesperidin shows potential as a
therapeutic candidate for treating type 2 diabetes and its associated
complications.
References:
[1]
Manna, P., & Jain, S. K., 2015, Obesity,
Oxidative Stress, Adipose Tissue Dysfunction, and the Associated Health Risks:
Causes and Therapeutic Strategies. Metabolic Syndrome and Related Disorders,
13(10), 423–444. https://doi.org/10.1089/met.2015.0095.
[2]
Bindu Jacob, & Narendhirakannan, R.T.,
2019, Role of medicinal plants in the management of diabetes mellitus: a
review. 3 Biotech, 9(1), 4. https://doi.org/10.1007/s13205-018-1528-0.
[3]
Kim, J., Wie, M. B., Ahn, M., Tanaka, A.,
Matsuda, H., & Shin, T., 2019, Benefits of hesperidin in central nervous
system disorders: a review. Anatomy & cell biology, 52(4), 369–377.
https://doi.org/10.5115/acb.19.119.
[4]
Giannini, I., Amato, A., Basso, L., Tricomi,
N., Marranci, M., Pecorella, G., Tafuri, S., Pennisi, D., & Altomare, D.
F., 2015, Flavonoids mixture (diosmin, troxerutin, hesperidin) in the treatment
of acute hemorrhoidal disease: a prospective, randomized, triple-blind,
controlled trial. Techniques in Coloproctology, 19(6), 339–345.
https://doi.org/10.1007/s10151-015-1302-9.
[5]
Prasad, M., Rajagopal, P., Devarajan, N.,
Veeraraghavan, V. P., Palanisamy, C. P., Cui, B., Patil, S., & Jayaraman,
S., 2022, A comprehensive review on high -fat diet-induced diabetes mellitus:
an epigenetic view. The Journal of Nutritional Biochemistry, 107,
109037. https://doi.org/10.1016/j.jnutbio.2022.109037.
[6]
Georgel, P. T., & Georgel, P. (2021). Where
Epigenetics Meets Food Intake: Their Interaction in the Development/Severity of
Gout and Therapeutic Perspectives. Frontiers in Immunology, 12, 752359.
https://doi.org/10.3389/fimmu.2021.752359.
[7]
Drabkin, D.L., Austin, J.H., 1932,
Spectrophotometric Studies: I. Spectrophotometric Constants for Common
Hemoglobin Derivatives in Human, Dog, and Rabbit Blood. Journal of
Biological Chemistry, 1932, 98, 719-733. 10.4236/oalib.1100648.
[8]
Bannon P., 1982, Effect of pH on the
elimination of the labile fraction of glycosylated hemoglobin. Clinical Chemistry,
28(10), 2183.
[9]
Slaoui, M., & Fiette, L., 2011,
Histopathology procedures: from tissue sampling to histopathological
evaluation. Methods in molecular biology (Clifton, N.J.), 691, 69–82.
https://doi.org/10.1007/978-1-60761-849-2_4.
[10]
Gabe, M., 1968, Techniques Histologiques, 6th
ed.; Massie e Cie: Paris, France, 1113p.
[11]
Slaoui, M., & Fiette, L., 2011,
Histopathology procedures: from tissue sampling to histopathological
evaluation. Methods in Molecular Biology (Clifton, N.J.), 691, 69–82.
https://doi.org/10.1007/978-1-60761-849-2_4.
[12]
Gancedo, J. M., & Gancedo, C., 1971,
Fructose-1,6-diphosphatase, phosphofructokinase and glucose-6-phosphate
dehydrogenase from fermenting and non fermenting yeasts. Archiv fur
Mikrobiologie, 76(2), 132–138. https://doi.org/10.1007/BF00411787.
[13]
Galicia-Garcia, U., Benito-Vicente, A., Jebari,
S., Larrea-Sebal, A., Siddiqi, H., Uribe, K. B., Ostolaza, H., & Martín,
C., 2020, Pathophysiology of Type 2 Diabetes Mellitus. International Journal
of Molecular Sciences, 21(17), 6275. https://doi.org/10.3390/ijms21176275.
[14]
Merz, K. E., & Thurmond, D. C., 2020, Role
of Skeletal Muscle in Insulin Resistance and Glucose Uptake. Comprehensive
Physiology, 10(3), 785–809. https://doi.org/10.1002/cphy.c190029.
[15]
Thomas, D. D., Corkey, B. E., Istfan, N. W.,
& Apovian, C. M., 2019,
Hyperinsulinemia: An Early Indicator of Metabolic Dysfunction. Journal
of the Endocrine Society, 3(9), 1727–1747.
https://doi.org/10.1210/js.2019-00065.
[16]
Wilcox G., 2005, Insulin and insulin
resistance. The Clinical Biochemist. Reviews, 26(2), 19–39.
[17]
Zhao, C., Yang, C., Wai, S. T. C., Zhang, Y., P
Portillo, M., Paoli, P., Wu, Y., San Cheang, W., Liu, B., Carpéné, C., Xiao,
J., & Cao, H., 2019, Regulation of glucose metabolism by bioactive
phytochemicals for the management of type 2 diabetes mellitus. Critical Reviews
in Food Science and Nutrition, 59(6), 830–847.
https://doi.org/10.1080/10408398.2018.1501658.
[18]
Stanley Mainzen Prince, P., &
Kamalakkannan, N., 2006, Rutin improves glucose homeostasis in streptozotocin
diabetic tissues by altering glycolytic and gluconeogenic enzymes. Journal
of Biochemical and Molecular Toxicology, 20(2), 96–102.
https://doi.org/10.1002/jbt.20117.
[19]
Latha, M., & Pari, L., 2003,
Antihyperglycaemic effect of Cassia auriculata in experimental diabetes and its
effects on key metabolic enzymes involved in carbohydrate metabolism. Clinical
and Experimental Pharmacology & Physiology, 30(1-2), 38–43.
https://doi.org/10.1046/j.1440-1681.2003.03785.x.
[20]
Peng, P., Jin, J., Zou, G., Sui, Y., Han, Y.,
Zhao, D., & Liu, L., 2021, Hesperidin prevents hyperglycemia in diabetic
rats by activating the insulin receptor pathway. Experimental and Therapeutic
Medicine, 21(1), 53. https://doi.org/10.3892/etm.2020.9485.
[21]
Pari, L., & Rajarajeswari, N., 2009,
Efficacy of coumarin on hepatic key enzymes of glucose metabolism in chemical
induced type 2 diabetic rats. Chemico-biological Interactions, 181(3),
292–296. https://doi.org/10.1016/j.cbi.2009.07.018.
[22]
Gothandam, K., Ganesan, V. S., Ayyasamy, T.,
& Ramalingam, S., 2019, Antioxidant potential of theaflavin ameliorates the
activities of key enzymes of glucose metabolism in high fat diet and
streptozotocin - induced diabetic rats. Redox report : Communications in free
radical research, 24(1), 41–50. https://doi.org/10.1080/13510002.2019.1624085.
[23]
Boucher, J., Kleinridders, A., & Kahn, C.
R., 2014, Insulin receptor signaling in normal and insulin-resistant states. Cold
Spring Harbor Perspectives in Biology, 6(1), a009191. https://doi.org/10.1101/cshperspect.a009191.
[24]
Zhang Y, Xu W, Huang X et al., 2018,
Fucoxanthin ameliorates hyperglycemia, hyperlipidemia and insulin resistance in
diabetic mice partially through IRS-1/PI3K/Akt and AMPK pathways. Journal of
Functional Foods, 48:515–524. 10.1016/j.jff.2018.07.048.
[25]
Cai, S., Sun, W., Fan, Y., Guo, X., Xu, G., Xu,
T., Hou, Y., Zhao, B., Feng, X., & Liu, T., 2016, Effect of mulberry leaf
(Folium Mori) on insulin resistance via IRS-1/PI3K/Glut-4 signalling pathway in
type 2 diabetes mellitus rats. Pharmaceutical Biology, 54(11),
2685–2691. https://doi.org/10.1080/13880209.2016.1178779.
[26]
Wang, Y., Nishina, P. M., & Naggert, J. K.,
2009, Degradation of IRS1 leads to impaired glucose uptake in adipose tissue of
the type 2 diabetes mouse model TALLYHO/Jng. The Journal of Endocrinology,
203(1), 65–74. https://doi.org/10.1677/JOE-09-0026.
[27]
Li, H., Yu, L., & Zhao, C., 2019, Dioscin
attenuates high‑fat diet‑induced insulin resistance of adipose tissue through
the IRS‑1/PI3K/Akt signaling pathway. Molecular Medicine Reports, 19(2),
1230–1237. https://doi.org/10.3892/mmr.2018.9700.
[28]
Ramalingam, K., Yadalam, P. K., Ramani, P., Krishna, M., Hafedh, S.,
Badnjević, A., Cervino, G., & Minervini, G., 2024, Light gradient
boosting-based prediction of quality of life among oral cancer-treated
patients. BMC Oral Health, 24(1), 349. https://doi.org/10.1186/s12903-024-04050-x.
[29] Neralla, M.,
M, H., Preethi, A., Selvakumar, S. C., & Sekar, D., 2024, Expression levels
of microRNA-7110 in oral squamous cell carcinoma. Minerva Dental and Oral
Science, 73(3), 155–160. https://doi.org/10.23736/S2724-6329.23.04801-5.
[30] Ramsundar,
K., Jain, R. K., Balakrishnan, N., & Vikramsimha, B., 2023, Comparative
evaluation of bracket bond failure rates of a novel non-primer adhesive with a
conventional primer-based orthodontic adhesive - a pilot study. Journal of Dental Research, Dental Clinics,
Dental Prospects, 17(1),
35–39. https://doi.org/10.34172/joddd.2023.36953.